All published articles of this journal are available on ScienceDirect.
Polymerase Chain Reaction in Intraocular Inflammation
Abstract
Polymerase chain reaction (PCR) is a technique involving enzymatic amplification of nucleic acid sequences in repeated cycles of denaturation, oligonucleotide annealing and DNA polymerase extension. It is a powerful molecular biologic tool that allows the rapid production of analytic quantities of DNA from small amounts of starting material. PCR can be performed on nearly any ocular specimen or biopsy. For diagnosis of uveitis, the obtained sample is usually an anterior chamber paracentesis or vitreous tap. PCR potentially is more sensitive than culture for detection of many organisms. By utilizing a secondary detection system in concert with the initial PCR reaction, perfect specificity can be assured. The initial application of PCR diagnostics to ophthalmic disease was in the detection of viral uveitis. PCR has also been implicated in studies of noninfectious uveitis. The most common application is HLA typing. A universal bacterial PCR can be very helpful for the diagnosis of bacterial endophthalmitis at an early stage of the disease.
INTRODUCTION
The polymerase chain reaction (PCR) is a powerful molecular biologic tool that allows the rapid production of analytic quantities of DNA from small amounts of starting material. Since the introduction of its modern form in 1988 [1], PCR has revolutionized much of molecular biology and has greatly accelerated the development of molecular diagnostics. Kary B. Mullis from USA received a Nobel Prize in 1993 for inventing this technique. This powerful technique has numerous applications in diagnostic pathology, especially in the fields of microbiology and genetics. All practicing ophthalmologists should have a working knowledge of the uses of PCR. PCR has been used to diagnose uveitis, infectious endophthalmitis and protozoal eye diseases [2]. This review discusses the use of PCR in the analysis of uveitis, and ways in which PCR is improving our knowledge of understanding of the mechanisms of uveitis.
BASIC TECHNIQUE
Polymerase chain reaction (PCR) is a technique involving enzymatic amplification of nucleic acid sequences in repeated cycles of denaturation, oligonucleotide annealing and DNA polymerase extension [3].The PCR uses in vitro enzymatic synthesis to amplify specific DNA sequence within few hours. The PCR consists of repetitive cycles of specific DNA synthesis, defined by short stretches of preselected DNA. With each cycle there is a doubling of the final, desired DNA product such that million-fold amplification is possible [4].
PCR is performed using two specific primers that flank the DNA region of interest. After enzymatic synthesis of the replicated strand is complete, the DNA is denatured into single strands. This allows the newly synthesized strand to serve as template for subsequent synthesis of new strands. Using an automated thermal heat block, 30 to 40 rounds of replication can be performed in just a few hours. Theoretically, the molar amount of PCR product doubles with each round of replication. Thirty-five cycles are typically used for diagnostic PCR. In order to perform PCR, we must have a source of DNA (DNA extracted either from an aqueous or vitreous specimen). It begins with the initial sample containing the target DNA and mixes in the appropriate primers, DNA polymerase, nucleotide triphosphates, and buffered salts. Following performance of PCR in the thermal cycler, the products may be detected in one of several ways. Generally, gel electrophoresis, with use of acrylamide or agarose, is employed to determine if a DNA fragment of expected size has been produced. Confirmation of the identity of the PCR product can be achieved by digesting the product with restriction endonuclease and observing the restriction digest pattern, a technique called fingerprinting. Ultimate identification of a DNA fragment can be achieved by sequencing the PCR product DNA.
Real-time polymerase chain reaction, also called quantitative real time polymerase chain reaction (QRT-PCR) or kinetic polymerase chain reaction is used to determine whether or not a specific sequence is present in the sample; and if it is present, the number of copies in the sample. It is the real-time version of quantitative polymerase chain reaction (Q-PCR), itself a modification of polymerase chain reaction.
The polymerase chain reaction is an effective tool for amplifying DNA, however for this to be adapted to measure RNA, the RNA sample first needs to be reverse transcribed to DNA via an enzyme known as a reverse transcriptase. This transcribed DNA is known as cDNA or complementary DNA. This method, known as RT-PCR, required extensive optimisation of the number of PCR cycles, so as to obtain results during logarithmic DNA amplification. Nested polymerase chain reaction is a modification of polymerase chain reaction intended to reduce the contaminations in products due to the amplification of unexpected primer binding sites. Nested polymerase chain reaction involves two sets of primers, used in two successive runs of polymerase chain reaction, the second set intended to amplify a secondary target within the first run product.
PCR can be performed on nearly any ocular specimen or biopsy. For diagnosis of uveitis, the obtained sample is usually an anterior chamber paracentesis or vitreous tap. Anterior chamber paracentesis of 50 microlitres is usually sufficient for diagnostic purposes. For vitrectomy specimens, the initial preinfusion aspirate (100-500 microlitre) is preferred. Specimens should be aseptically transferred to a sterile, capped tube (i.e., a 1.5-ml microfuge tube) and quick-frozen on dry ice or in liquid nitrogen. The sample should remain frozen until processed by the accepting laboratory; freeze thaw cycles will release nucleases that will degrade all RNA and some DNA [5].
The sensitivity for detection of foreign DNA is very high. Thus PCR potentially is more sensitive than culture for detection of many organisms. By utilizing a secondary detection system in concert with the initial PCR reaction, perfect specificity can be assured. Although PCR would seem to have nearly ideal characteristics for a diagnostic test, the high sensitivity and specificity can cause significant pitfalls.
PCR DIAGNOSIS OF INFECTIOUS UVEITIS
PCR has had a major impact on our ability to detect infectious agents. Since the first detection of Toxoplasma gondii DNA in ocular tissue with the use of PCR in 1990 [6]. PCR has been applied to the detection and diagnosis of various infective uveitis [7].
The initial application of PCR diagnostics to ophthalmic disease was in the detection of viral uveitis [5, 8-10]. Knox et al. [10] performed PCR on aqueous or vitreous samples of 37 eyes of 38 patients, with “diagnostic dilemmas” in posterior uveitis. Of these cases, a definitive diagnosis of a viral infection could be made by PCR in 25 eyes. Of the PCR-negative cases, a number were ultimately diagnosed to be toxoplasmosis, and the remainder had natural histories inconsistent with viral retinitis. Thus, both positive and negative PCR results likely had diagnostic significance in this study. Probably the most common indication for performing diagnostic PCR for posterior uveitis is the presence of media opacity. Significant media opacity from cataract or dense vitritis can make otherwise straightforward diagnoses difficult. Mitchell et al. developed PCR primers with a sensitivity of 93% and specificity of 98% for the detection of Cytomegalovirus (CMV) [11]. Of the nine patients tested, four tested positive for CMV, and three for Varicella Zoster virus (VZV). The remaining two were subsequently judged to have toxoplasmosis. In all cases, the clinical course was consistent with the PCR-based diagnosis. The clinical diagnosis of atypical toxoplasmosis can also be problematic. Classical reactivation toxoplasmosis can be diagnosed by clinical examination, but primary toxoplasmosis can resemble a number of other infectious acute retinitis [12, 13]. Initial studies of PCR diagnosis of Toxoplasma gondii were disappointing, showing sensitivities less than 50% [14, 15]. In 1993, Aouizerate et al. performed PCR on the aqueous of 59 eyes with suspected or confirmed infection with Toxoplasma gondii; the parasite was demonstrated in 20 cases (33.8%) [14]. However, recent advances in primer design, utilizing highly repetitive pathogen DNA sequences, have greatly improved yields for PCR of T. gondii. Montoya et al. [15] were able to detect Toxoplasma DNA in nearly 80% of patients with suspected ocular toxoplasmosis and positive serum IgG titers. Using a similar PCR assay, Bou et al. [16] were able to detect Toxoplasma gondii DNA in the peripheral blood of most patients with active ocular toxoplasmosis, raising the possibility that in the future, reactivation disease could be diagnosed via a blood test. Biswas et al. performed PCR on the aqueous in a case of suspected miliary tuberculosis of choroids (Fig. 1) and Mycobacterium tuberculosis genome (Fig. 2) was found in PCR analysis [17].PCR is also helpful in detecting Leptospira related uveitis [18].
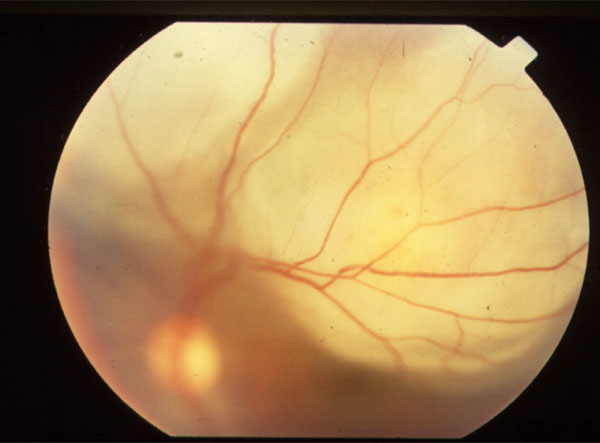
A case of Subretinal abscess.
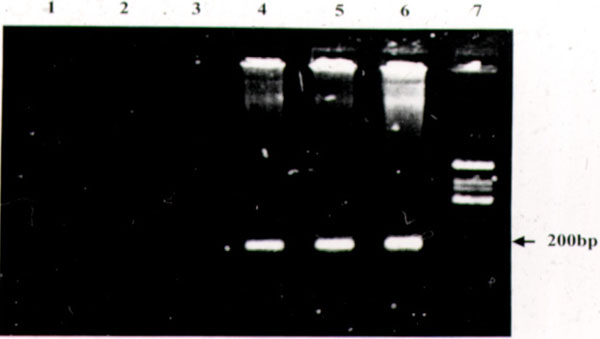
Ethidium bromide stained 2% agarose gel with amplification products from a case of subretinal abscess suspected to be tuberculous. Lane 1: Reagent control of the first round. Lane 2: Reagent control of the second round. Lane 3: Aqueous humor - negative, Lane 4: FNAB specimen - positive, Lane 5: Blood - positive. Lane 6: Positive control M. tuberculosis (H37Rv). Lane 7: Phi x 174 DNA/Hinf l digest.
PCR IN RETINAL VASCULITIS
Madhavan et al. reviewed their experience using PCR to tissue sections obtained from formalin-fixed and paraffin embedded tissues of epiretinal membrane (ERM) from 23 patients of Eales’ disease [19]. 11 out of 23 (47.8%) were positive for Mycobacterium tuberculosis genome, indicating association of this bacterium with Eales’ disease. Gupta A et al. reported tubercular retinal vasculitis with varied fundus findings, and diagnosis was confirmed by doing PCR from the aqueous or vitreous humor [20].
PCR DIAGNOSIS OF NONINFECTIOUS UVEITIS
PCR has also been implicated in studies of noninfectious uveitis. The most common application is HLA typing. Saiki et al. used PCR to enzymatically amplify a specific segment of beta-globin or HLA-DQ alpha gene in human genomic DNA [21].
Polymerase chain reaction-restriction fragment length polymorphism (PCR-RFLP) methodology is applied to HLA-DR, -DQ and -DW typing at the nucleotide level, eliminating the need for radioisotopes as well as allele specific oligonucleotide probes [22].
Using this technique, Shino et al. reported complete association of the HLA-DRB104 and -DQB104 alleles with Vogt-Koyanagi-Harada (VKH) disease [23].and it is more common for Asians. Polymerase chain reaction-sequencing-based typing (PCR-SBT) is used for HLA-B51 alleles. Evaluation of intraocular cytokines and other inflammatory mediators and makers provides important information, particularly in noninfectious uveitis [24]. Cytokines and inflammatory related transcripts are usually detected via reverse transcription PCR (RT-PCR) [24-26].The results from RT-PCR are complementary to data from Western blotting and/or immunohistochemistry.
PCR FOR MASQUERADE SYNDROME
The Masquerade syndrome is consisting of group of disorders that occurs with intra-ocular inflammation (most commonly malignancy) and is often misdiagnosed as uveitis. PCR can be useful for diagnosing masquerade syndrome. Primary intraocular lymphoma is a subtype of central nervous system lymphoma involving the eye. It can often mimic chronic uveitis. Utilization of PCR has become a practical tool for the detection of IgH gene rearrangements and provides a helpful adjunct for the diagnosis of B-cell lymphoma in the eye [27].
PCR FOR ENDOPHTHALMITIS
Although direct microscopy is the easiest and most rapid method to detect bacterial etiologies of endophthalmitis, its sensitivity is very low, with positive result varying from 4.2% to 46.5% for vitreous samples, which decreases further in aqueous fluid [28, 29]. More sensitive than microscopy, culture is considered “ the gold standard”. However, there have been no significant improvements in the yield of culture methods [30]. Postoperative endophthalmitis is a vision-threatening complication of cataract surgery and presents even further diagnostic challenges. The organisms are frequently present in low numbers, and they can be difficult to culture. Yields from diagnostic vitreous biopsies in this condition are less than 50%. The Endophthalmitis Vitrectomy Study reported culture yields of only 70% [31]. Culture results are also slow to return, thus requiring patients be treated with broad-spectrum antibiotics for several days, even for relatively indolent bacteria. In cases where conventional techniques have low sensitivity, PCR, characterized by its high sensitivity and specificity, would be an ideal technique to detect bacterial pathogens in the eye. All bacteria share common, highly repetitive DNA sequences for their 16S ribosomal RNA. By designing primers to these conserved 16S sequences, PCR can be performed on biopsy material from eyes with suspected endophthalmitis, with the results available within 6-8 hours. Therese et al. demonstrated the utility of this approach for culture-negative endophthalmitis [29]. They were able to determine a bacterial cause for endophthalmitis in 100% of culture-positive and 44% of culture-negative cases. Of the remaining culture-negative cases, one-third was found to be fungal. Lohmann et al. [32]. used 16S ribosomal primers as well as fungal PCR primers, along with culture and stain for 25 eyes with delayed-onset endophthalmitis. Aqueous culture and microscopy each had a 0% yield, but vitreous culture had a 24% yield in these patients. PCR of the aqueous yielded a diagnosis in 84% of the cases and PCR of a vitreous biopsy yielded a diagnosis in 92%. PCR thus has clear superiority to any other available diagnostic technique for diagnosis of endophthalmitis.
Biswas et al. demonstrated Aspergillus fumigatus fungus by PCR-based RFLP (Restriction Fragment Length Polymorphism) technique from paraffin section of an eyeball of an eight-month-old child removed for endogenous endophthalmitis [33].
Compared to the conventional technique, PCR for detection of fungal DNA was found to be a rapid and more sensitive method in the early diagnosis of postoperative fungal Endophthalmitis [34, 35]. Semi-nested polymerase chain reaction is also helpful for rapid detection of panfungal genome directly from ocular specimens [36].
Polymerase chain reaction-based technology is a useful adjunct to conventional culture because when used with aqueous humor samples only, the association of both techniques allowed for a microbiological diagnosis in 71% of cases of postoperative acute and delayed-onset endophthalmitis [37]. A universal bacterial PCR can be very helpful for the diagnosis of endogenous bacterial endophthalmitis at an early stage of the disease [38].
CONCLUSION
PCR is a powerful molecular technique for evaluation of very small amounts of DNA and RNA. PCR can be a simple, rapid, sensitive and specific tool for the diagnosis of infection, autoimmunity and masquerade syndromes of the eye.