All published articles of this journal are available on ScienceDirect.
Concentration of Droplets from Patients during Normal Breathing and Speech and Their Importance in Protection from Coronavirus SARS-CoV-2 (COVID-19) Infection
Abstract
Purpose:
Coronavirus disease (COVID-19) has been declared a pandemic and the number of infected individuals and deaths continue to increase globally. COVID-19 is transmitted through airborne droplets formed during coughing and sneezing and from the saliva of infected patients. Medical healthcare workers are often at risk of infection. This study measured the aerosol derived from the droplets of patients during the conversation.
Methods:
Overall, 25 patients aged 21 to 87 years were enrolled. The amount of droplets from the patient was measured under the following four conditions: 1) no conversation with the mask on; 2) conversation with the mask on; 3) conversation without the mask; and 4) no conversation without the mask. Particulate matter (PM) with an aerodynamic diameter of 2.5 μm or less (PM2.5) and PM with a diameter of 10 μm or less (PM10) were measured as representative aerosols at a position of 1 meter from the patients.
Results:
The concentrations of PM2.5 (µg/m3) were as follows: 22.7 ± 10.2 before the conversation with the mask, 24.2 ± 10.2 during the conversation with the mask, 32.3 ± 14.7 during the conversation without the mask, and 23.1 ± 9.9 after the conversation without the mask. The concentrations of PM10 (µg/m3) were as follows: 39.8 ± 18.2 before conversation with the mask, 41.9 ± 18.5 during conversation with the mask, 55.5 ± 27.2 during conversation without the mask, and 40.4 ± 17.8 after conversation without the mask. The variations in the PM2.5 and PM10 correlated negatively with the age of patients (PM2.5: r = -0.51, p = 0.0009 and PM10: r = -0.53, p = 0.0063).
Conclusion:
Wearing a mask can prevent airborne droplet formation and reduce transmission of infection.
1. INTRODUCTION
Coronavirus disease (COVID-19) is an infectious disease caused by the severe acute respiratory syndrome coronavirus 2 (SARS-CoV-2). The World Health Organization (WHO) declared its outbreak a pandemic on March 11, 2020. Healthcare workers are at the frontline of the risk of infection and death caused by the outbreak. In fact, many health professionals have been infected and have died since the wake of the outbreak worldwide. Moreover, its rapid spread has put them under increasing work-related stress and mental health risks.
As per WHO, the potential mode of transmission for SARS-CoV-2 is through respiratory droplets and contact routes. Asymptomatic COVID-19 carrier individuals can also transmit infection. According to recent reports, SARS-CoV-2 gets transmitted between people mainly via close contact within 1 m and respiratory droplets through sneezing and coughing [1-6]. However, to date, airborne transmission of COVID-19 has not been reported. Ong and associates reported no airborne transmission in an analysis of 75,465 COVID-19 cases in China [7]. The results of these analyses have been cited as strong evidence that the COVID-19 virus is mainly transmitted through direct person-to-person contact.
A respiratory droplet is often defined as a droplet with >5-10 μm diameter that falls rapidly to the ground under gravity after being produced by breathing, talking, sneezing and coughing [8, 9]. Therefore, these droplets are transmitted only over a limited distance, generally ≤1 m. In contrast, the term droplet nuclei often refers to droplets with ≤5 μm diameter that can remain suspended in the air at least for hours, in a dry state and can be transmitted over distances >1 m [10, 11]. These transmitted droplet nuclei can be inhaled into the lower respiratory tract. But, so far, it is not known about the concentration of droplets generated when an infected person takes a breath or talks. This is important as it will help in understanding the dynamics of transmission of SARS-CoV-2. In this study, we investigate the concentration of droplets emitted by patients during conversation.
2. METHODS
2.1. Research Design and Participants
This was a prospective non randomised uncontrolled before and after trial of adult participants. It was performed in accordance with the Declaration of Helsinki and was approved by the Ethics Committee of Teikyo University as a study on the causative bacteria of ocular infections (#Teirin 18-227) and performed at the outpatient clinic of Teikyo University Hospital. The present study was registered as UMIN000039595 by the University Hospital Medical Information Network Clinical Trials Registry (UMIN-CTR) in Japan. Twenty-five patients were enrolled consecutively from an ophthalmology outpatient clinic from April 2020 to May 2020. Informed consent was obtained from each of them. Their mean age was 59.8 ± 20.0 years, with a range of 21-87 years and included 9 women and 16 men. No patients had respiratory symptoms or coughing problem.
2.2. Measurement of Droplets from Patients
The amount of salivary droplets generated from the patient's mouth was measured during the face-to-face examination of the patient. The patients were fully informed about the study and its procedures prior to the test. The measurements were performed in the following four states: (1) No conversation with mask on, (2) Conversation with mask on, (3) Conversation without the mask, and (4) No conversation with mask off. All patients wore their own disposable non-woven masks. Fine particulate matter with an aerodynamic diameter of 2.5 μm or less (PM2.5) and particulate matter with an aerodynamic diameter of less than 10 μm (PM10) were measured as a surrogate parameter for the droplets. An interval of 3-5 minutes was set after each measurement.
We measured both PM2.5 and PM10 concentrations using a digital air quality detector (SMART SENSOR 5-in-1, KKmoon from Shenzhen Tomtop Technology Co., Ltd, Shenzhen, Guangdong, China). The device was installed at a distance of 1-meter from the patients. The room had a size of 4 m × 3 m and the height of the room was approximately 3 m. Patients sat on chairs at least 1 m away from the wall. The room temperature was set between 20-22 degree with no special humidity control. The measured values were recorded and saved in a Microsoft Excel® file (ver. Microsoft Office 365, Microsoft Corporation, Redmond, WA, USA) on the computer.
2.3. Statistical Analysis
One-way analysis of variance and the Tukey-Kramer multiple comparison test were used to compare the mean values among the four groups. Relations among variables were investigated by calculating the Pearson correlation coefficients and partial correlation coefficients. Statistical analyses were performed with SAS System software version 9.1 (SAS Institute Inc., Cary, NC, USA), and significance was accepted at p < 0.05.
3. RESULTS
The concentration of PM2.5 was 22.7 ± 10.2 µg/m3 before the conversation with the mask, 24.2 ± 10.2 µg/m3 during conversation with the mask, 32.3 ± 14.7 µg/m3 during conversation without the mask, and 23.1 ± 9.9 µg/m3 after conversation without the mask (Fig. 1).
The concentration of PM10 was 39.8 ± 18.2 µg/m3 before conversation with the mask, 41.9 ± 18.5 µg/m3 during conversation with mask, 55.5 ± 27.2 µg/m3 during conversation without the mask, and 40.4 ± 17.8 µg/m3 after conversation without the mask (Fig. 2).
There were significant differences observed among the four groups in the PM2.5 (p = 0.0106) and PM10 (p = 0.0254), and the highest values of PM2.5 and PM10 were observed in the group without a mask during the conversation (one-way analysis of variance and the Tukey-Kramer multiple comparison test).
Next, we calculated the difference in the concentration of PM2.5 (variation of PM2.5) or PM10 (variation of PM10) before conversation with the mask and during conversation without a mask. A significant correlation was observed between variation of PM2.5 and PM10 (r = 0.97, p < 0.0001, Pearson product moment correlation coefficient) (Fig. 3).
Variations in both PM2.5 and PM10 were negatively correlated with the age of patients (r = -0.51, p = 0.0009 and r = -0.53, p = 0.0063, respectively, Pearson product moment correlation coefficient) (Fig. 4).
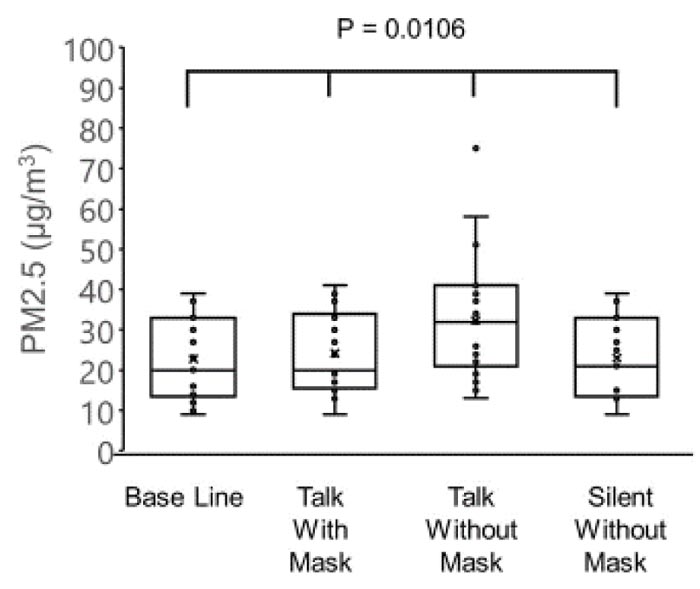
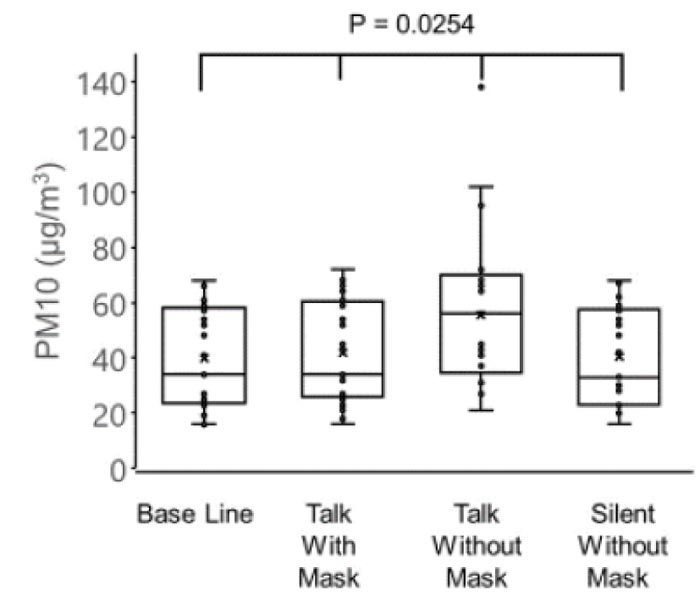
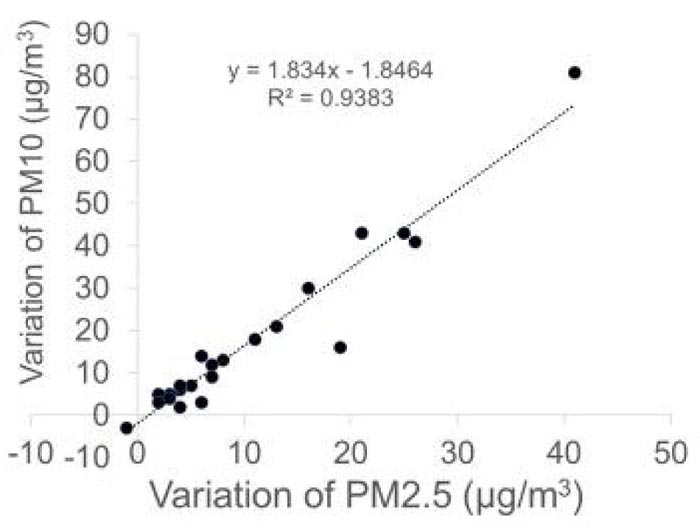
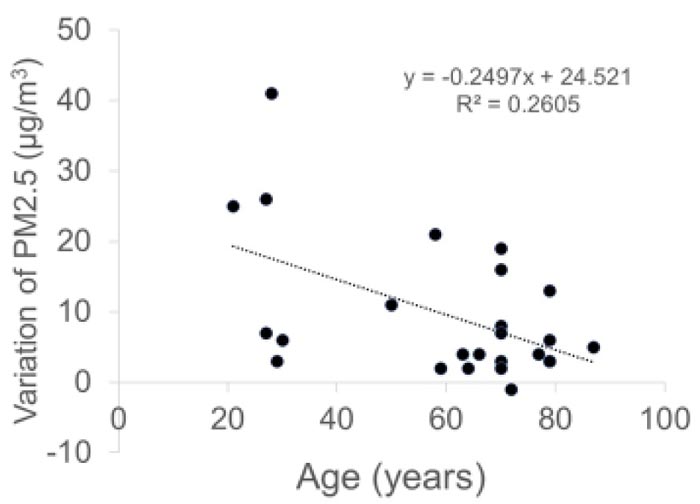
4. DISCUSSION
This study first demonstrated the presence of droplets from the patient's mouth in the ophthalmic outpatient clinic. There are two important points as a result, one is that the amount of droplets can be dramatically reduced by wearing a mask, and the second is that the droplet volume decreases with age.
Generally, large droplets fall quickly to the ground. According to a study by Morawska and associates, droplets of 1000 µm, 100 µm, 10 µm, and 1 µm take 0.3 seconds, 3 seconds, 300 seconds, and 30,000 seconds, respectively, to fall at a distance of 1 meter [12, 13]. That is, these results indicate that the droplets will probably drop to the ground from over 1 meter of the patients [12-14]. Therefore, in this study, measurement was performed at a distance of 1 meter from the patients. Additionally, Yang and associates reported that the size distribution of droplet nuclei coughed by the test subjects ranged from 0.58-5.42 µm, and 82% of the droplet nuclei had a size in the range of 0.74-2.12 µm [15]. The size distribution of the coughed droplets was 0.62-15.9 µm, with an average size of 8.35 µm [15]. Therefore, we used PM10 as an index of droplets, and PM2.5 as an index of droplet nuclei in this study. Even in the steady-state condition of the room, droplets are floating in the air, which is equivalent to the concentration of PM2.5 and PM10 before the conversation with mask in this study. In contrast, the difference in PM2.5 or PM10 measured before and during the conversation can be considered to be the amount of droplets released from the patient. Our results showed that both PM2.5 and PM10 from a patient during conversation were suspended in the air at a distance of 1 meter from the patient. Generally, droplets > 5 μm in diameter fall to the ground within 1 meter under gravity [9]. In contrast, droplet nuclei ≤ 5 μm in diameter can remain suspended in the air and be transmitted over distances > 1 m [9-11]. In the current study, a modest amount of PM10 was detected, even at one meter from the patients. If we measured at a closer distance, around 50 cm from the patients, a higher concentration of PM10 would have been detected. Incidentally, PM10 is defined by ISO as a particulate matter which passes through a size-selective inlet that has a 50% efficiency cut-off at 10 μm aerodynamic diameter. Therefore, the amount of PM10 we measured does not include PM2.5 particles.
Thus, droplets can travel to a distance of over 1 meter from the patients if the patient talks without wearing a mask. Face-to-face consultation between a patient and a doctor at a distance of 1 meter is quite common in any department; however, our results clearly suggest that we need to have sufficient distance from the patient while talking. Further, we would like to emphasize that wearing a mask by the patient can significantly prevent splashes. This fact suggests that both doctors and patients need to wear masks to prevent unknown infections.
Interestingly, the amount of droplets from patients decreased with the patients’ age. The concentration of droplets and droplet nuclei may be related to the saliva volume and respiratory function of each patient. The total volume of saliva produced in a healthy person each day is approximately 1,000-1,500 ml [16], and it is generally accepted that saliva production gradually decreases with age in adults [17, 18]. The respiratory function also declines with age in adults [19]. As a result, the amount of droplets is more in healthy young people who produce a large amount of saliva and have a higher respiratory function. Additionally, their tiny droplets of saliva can travel a greater distance very quickly along the stream of breath.
This study has several limitations. First, in this study, droplets were measured only at a distance of 1 meter. In the future, data on droplet concentrations at other distances will also be useful for people of other occupations. Additionally, measuring droplets at the position of the slit lamp will provide useful information for ophthalmologists. Second, we did not investigate whether there is a difference in the concentration of droplets that pass through the mask depending on the type of mask. In the future, it will be necessary to find out this difference using other types of masks, such as surgical and N95 masks. In this study, all patients wore their own disposable masks. Third, we found that the droplet concentration decreased with age. However, we did not investigate the relationship between droplet concentration and physique (patients’ height and weight) or respiratory function.
CONCLUSION
We found that droplets and droplet nuclei are generated from the patient's mouth during the examination of the patient. Furthermore, we found that the concentration of the droplets is higher in younger patients, and that the droplet exposure can be prevented by wearing a mask. Various unknown viruses, such as SARS-CoV-2, may be prevalent in hospitals. We need to educate patients to wear masks in clinics and hospitals to protect themselves and healthcare workers from acquiring such easily transmissible infections.
ETHICS APPROVAL AND CONSENT TO PARTICIPATE
This study was approved by the Ethics Committee of Teikyo University as a study on the causative bacteria of ocular infections (#Teirin 18-227) and has been performed at the outpatient clinic of Teikyo University Hospital.
HUMAN AND ANIMAL RIGHTS
No animals were used in this research. All human research procedures followed were in accordance with the ethical standards of the committee responsible for human experimentation (institutional and national), and with the Helsinki Declaration of 1975, as revised in 2013.
CONSENT FOR PUBLICATION
Informed consent was obtained from each of the participants.
AVAILABILITY OF DATA AND MATERIALS
Not applicable.
FUNDING
This work was supported in part by a Grant-in-Aid for Scientific Research from the Ministry of Education, Culture, Sports, Science and Technology of Japan (16K11332).
CONFLICT OF INTEREST
The authors report no conflicts of interest. The authors alone are responsible for the content and writing of this paper.
ACKNOWLEDGEMENTS
Declared none.