All published articles of this journal are available on ScienceDirect.
Cell Adhesion Molecule Expression in Human Lens Epithelial Cells After Corticosteroid Exposure
Abstract
Aim:
The aim of the study was to investigate changes in cell adhesion molecule expression in human lens epithelial cells (HLEC) subjected to glucocorticoids.
Methods:
Human lens epithelial cells were exposed to different concentrations of dexamethasone for 24 hours. Cell adhesion molecule expression was studied by western blot and immunohistochemistry of vimentin, N-cadherin, E-cadherin, α-catenin, β-catenin and γ-catenin. Expression of the glucocorticoid receptor (GR) was also studied. Cell morphology was examined by transmission electron microscopy (TEM).
Result:
Expression of N-cadherin, α-catenin, β-catenin and GR was significantly decreased in dexamethasone exposed cells as compared to unexposed cells. No significant change in γ-catenin was present. Visualization of adhesion molecules, N-cadherin and α-catenin, by immunohistochemistry showed decreased antigen reactivity in dexamethasone exposed as compared to the unexposed cells. However, no change was seen for β-catenin and γ-catenin. E-cadherin was not detectable using western blot or immunohistochemistry.
TEM showed multilayering of cells, vacuole formation and appearance of electron-dense multivesicular bodies in HLEC exposed to 0, 0.1, 1, 10 and 100 αM dexamethasone.
Conclusion:
Glucocorticoids affect several adhesion molecules in lens epithelial cells, something that may contribute to the pathogenesis of posterior subcapsular opacification.
INTRODUCTION
Glucocorticoid-induced cataract is a common clinical condition. Although the association between topical and systemic steroid use and increased occurrence of posterior subcapsular cataract (PSC) has been known since 1960 [1], the mechanism behind this type of lens opacification has yet not been elucidated. Over the years, several mechanisms such as osmotic failure [2, 3], metabolic disturbances [3], protein adduct formation [3-6], conformational changes due to oxidative stress [3, 6-8], and receptor mediated changes [3, 6, 8-11] have been proposed to account for PSC formation, but the results are inconclusive.
In recent years, aberrant cell migration, disrupted cell proliferation and differentiation, common features in PSC [12, 13], have been proposed to be due to perturbation of gene expression [14], reduction in E-cadherin [13] as well as the alteration of normal growth factor levels [3, 6].
Adherens junctions are protein complexes that form strong cell-cell contacts between adjacent cells. The classical view is that adherens junctions mediate adhesion between cells by linking the cytoplasmic tail of the calcium-dependent transmembrane protein, cadherin, directly to β- or γ-catenin, which binds to α-catenin that is connected to actin filaments. The stable intercellular adhesion provided by adherens junctions, maintain correct cell organization and homeostasis [15, 16]. Disruption of this stable cell attachment could lead to disturbed cell migration.
In cultured chick embryonic lens epithelial cells, N-cadherin has been shown to be localized to adherens junctions [17-19]. Studies have also shown that inhibition of N-cadherin leads to changes in expression of the cadherin-catenin complex as well as disrupted connection to the actin cytoskeleton [20].
Studies have suggested that glucocorticoids exert their actions both directly on lens function as previously mentioned, as well as indirectly via the glucocorticoid receptor (GR) [2, 4, 7, 8, 10, 11, 21-23]. In the lens, the presence of a functional GR was discussed for long. However, recent studies have demonstrated that the lens, including the human lens, has a classical functional GR [11, 24].
Glucocorticoids bind to GRs in the cytoplasm and the hormone-receptor complex is then translocated into the nucleus [23, 25, 26]. In the nucleus, the GC-GR complex binds to glucocorticoid response elements (GRE) in the target gene to activate or repress gene expression [11, 23, 26-28].
In a previous study from our group, we found an increase in proliferation as well as the apoptosis in native human lens epithelial cells after exposure to dexamethasone. The proapoptotic effect was not due to oxidative mechanisms, nor was it a GR-mediated effect [29].
In the present study, we have examined the effect of dexamethasone on cultured native human lens epithelial cells (HLEC) with respect to the adhesion molecule expression and GR-binding. Migration and morphology was investigated by western blot, immunohistochemistry and transmission electron microscopy.
MATERIALS AND METHODS
Human Lens Epithelial Cell Culture
Human lens epithelium specimens were obtained from lenses during cataract surgery at the Eye Clinic, Sahlgrenska University Hospital, Mölndal after informed consent. The study was approved by The Gothenburg University Ethics Committee and the tenets of the Declaration of Helsinki were followed. The human lens epithelium specimens, usually 5 mm in diameter, were put in eppendorf tubes containing culture medium (RPMI-1640), supplemented with 10% fetal calf serum, 100 U ml-1 penicillin, 0.1 mg ml-1 streptomycin and 2 mM L-glutamine immediately after surgery. The lens epithelium specimens were transferred from the eppendorf tubes to 24-well culture dishes (TPP, Switzerland) in a humified CO2-incubator at 37°C and the lens epithelial cells (HLEC) started to proliferate. When confluent, the HLEC were subcultured by 0.25% trypsin/EDTA treatment, followed by resuspension in medium RPMI-1640 with 10% fetal calf serum, 100 U ml -1 penicillin, 0.1 mg ml -1 streptomycin and 2 mM L-glutamine.
For each experiment, HLEC from two or more cell lines were used, each cell line containing cells from one individual, passage IV-VIII.
Electrophoresis, Western Blot
HLEC, exposed to 0 or 100 µM dexamethasone in serum-free culture medium for 24 hours was rinsed in ice cold PBS, followed by lysis in modified NuPage 0.5% lithium dodecyl sulphate (LDS) sample buffer (Invitrogen, Carlsbad USA).
The dexamethasone concentration used, was chosen according to dose response experiments of dexamethasone in a previous study from our group [29].
Whole cell lysate was used except for GR quantification where the cytoplasmic fraction was used. To separate the nucleus from the cytoplasm the cell lysate were centrifuged at 1200 g for 2 minutes. The cytoplasmic fraction was used for GR western blot analysis.
After subsequent heating of the cell lysate at 70°C for 10 minutes, protein concentration was determined using the BCA protein assay reagent (Pierce Perbio Science UK Limited, Cheshire, United Kingdom) with bovine serum albumin as standard. The samples were diluted to identical concentrations in NuPage LDS sample buffer and reducing agent (Invitrogen, Carlsbad USA) that was reduced to 10% of the total sample volume was added.
Equal amounts of protein (10 µg) were separated on precast NuPAGE Bis-tris 4-12% gradient minigels using NuPage MOPS as running buffer. For western blotting, proteins were transferred to nitrocellulose membranes followed by a blocking in 5% non fat milk powder (NFM) in phosphate buffered saline (PBS) for 1 hour.
Primary antibodies used for Western blotting included mouse monoclonal anti-E-cadherin (no. 610181) (1:5000), anti-N-cadherin (1:10 000), anti-α-catenin (1:500), anti-β-catenin (1:8000), anti-γ-catenin (1:8000) (all from BD Biosciences, USA), anti-vimentin (1:15 000) (Dako, Denmark), anti-β-actin (1:2000) (Sigma and polyclonal rabbit anti-GR (1:500) (Santa Cruz Biotechnology Inc., Europe).
For the adhesion molecule experiments, anti-vimentin was used as control. To detect small variations in gel loading between sample lanes in the GR experiments, anti-β-actin was used.
Primary antibody binding was detected with the corresponding secondary antibodies conjugated to horseradish peroxidase (Sigma Chemical, St Louis, MO USA).
Membranes were developed using the AmershamTM ECL Western blotting detection reagents (GE Healthcare, UK) and exposed to AmershamTM Hyperfilm ECL (GE Healtcare, UK), followed by densitometry analysis using ImageJ software version 1.37 (National Institute of Health, USA).
Immunohistochemistry
For immunohistochemistry labelling, cells were seeded in chamber slides (Lab-Tek™ Nalge Nunc International, Rochester, NY, USA). When semiconfluent, the cells were exposed to 0 or 100 µM dexamethasone for 24 hours followed by fixation in 4% paraformaldehyde solution (pH 7.4) for 15 minutes. The fixed and rinsed cells were preincubated in 5% NFM in phosphate buffered saline (PBS) for 30 min in room temperature. The cells were incubated with primary antibodies overnight at +4° C. Corresponding primary antibodies used in the western blot experiments were used at concentrations; mouse monoclonal anti-vimentin, (1:1000) anti-N-cadherin (1:100), anti-α-catenin (1:100), anti-β-catenin (1:100), anti-γ-catenin (1:100), rabbit polyclonal anti- GR (1:100), anti-E-cadherin (no. 610181, 610404), (1:100) (all from BD Biosciences, USA), anti-E-cadherin (1:100) (Invitrogen, Camarillo CA USA) and fluorescein isothiocyanate (FITC) conjugated phalloidin (1:100) (Sigma Chemical, St Louis, MO USA). After subsequent rinse in PBS, the cells were incubated with biotin-conjugated matching secondary antibodies for 1 hour at room temperature followed by incubation with streptavidin conjugated FITC (Vector Laboratories Burlingame, CA) according to standard immunohisto-chemistry protocol.
Further, cell nuclei were stained with Hoechst 33342 (Hoechst, Frankfurt Germany) at a final concentration of 5 μg ml −1 in a PBS buffer for 15 min. After being washed in PBS, the slides were mounted with Vectashield mounting media (Vector Laboratories Burlingame, CA) and immediately viewed using a fluorescence microscope (Nikon C1 Eclipse TE300; Nikon).
Transmission Electron Microscopy
HLEC cultured on a Transwell membrane were exposed to dexamethasone for 24 hours followed by fixation in Karnowsky fixative (2% paraformaldehyde, 2.5% glutaraldehyde, 0.01% sodium azide in 0.05 M sodium cacodylate) for 2 hours at 4° C. The cells were rinsed in 0.15 µM sodium cacodylate and postfixed in 1% osmium tetroxide (OsO4) and 1% potassium ferrocyanide (K4Fe(CN)6) for 2 hours at 4°C. Contrast was enhanced by 0.5% uranyl acetate for 1 hour in darkness at room temperature. After dehydration, the filters with attached cells were embedded in Agar 100 resin (Agar Scientific LTD, Essex, England). Ultrathin transverse sections (60-70 nm) of the filters with attached cells were contrasted with uranyl acetate and lead citrate before examination in a LEO 912AB transmission electron microscope (Carl Zeiss, Oberkochen, Germany). Digital image files were captured with a MegaView III camera (Soft Imaging Systems, Münster, Germany).
Statistics
Statistical analysis on densitometry results from western blot experiments was performed using independent-samples t-test. As software, SPSS 11.0.4 (SPSS Inc., Chicago, Il) were used. Experiments were run at least three times to confirm reproducibility and representative results are shown.
RESULTS
Effect of Dexamethasone on Protein Expression Quantified by Western Blot
Western blot and densitometry analysis showed decreased N-cadherin, α-catenin, β-catenin and GR expression in dexamethasone exposed HLEC as compared to the unexposed cells (Figs.1,2). The result indicates a decreased cellular adherens.
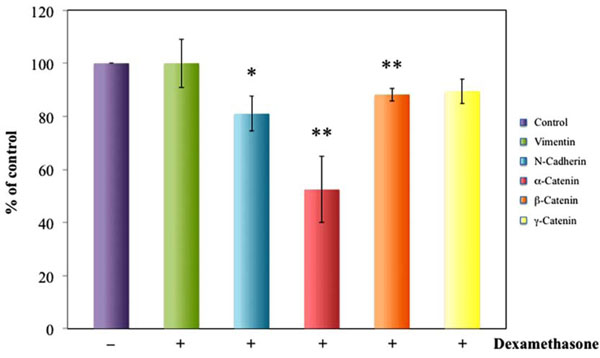
Densitometric analysis of western blot reveals significantly decreased N-cadherin α-catenin and β-catenin expression in HLEC exposed to dexamethasone for 24 hours as compared to unexposed cells. Dexamethasone exposure did not change γ-Catenin or vimentin expression. Vimentin was used as positive control for adhesion molecule detection. *P<0.05, **P<0.01.
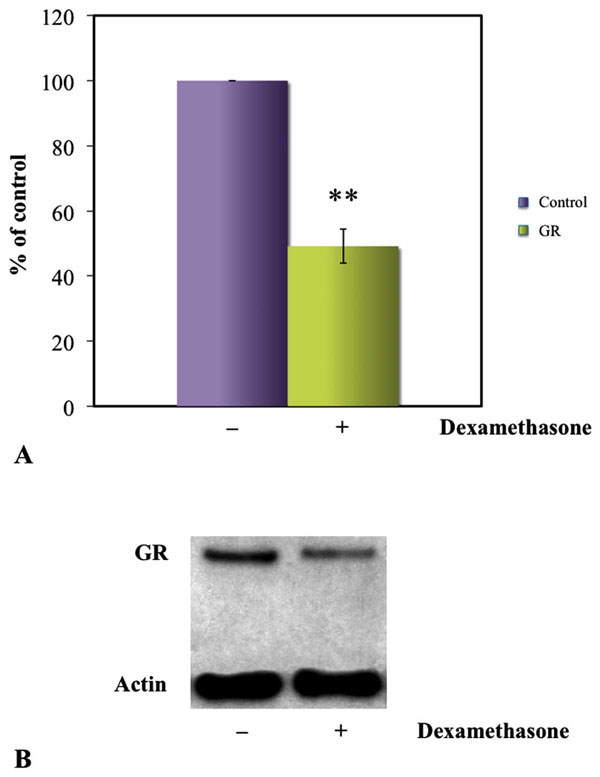
Densitometry of GR bands in western blots showed a significant decrease of GR in the cytoplasm of dexamethasone exposed cells as compared to the unexposed (A). Western blot of HLEC presenting significantly weaker GR expression in HLEC exposed to 100 µM dexamethasone as compared to unexposed cells. β-actin was used as loading control (B). *P<0.05.
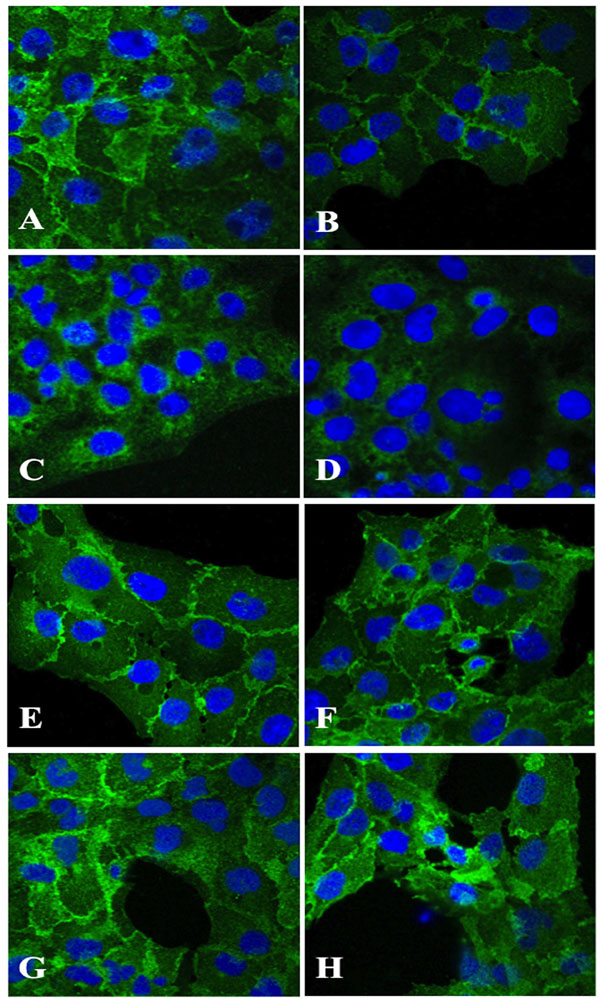
Immunohistochemical staining of HLEC exposed 100 µM dexamethasone (B, D, F, H) for 24 hours indicated a decline in antigen reactivity of N-cadherin (B) and α-catenin (D), whereas β-catenin (F) and γ-Catenin (H) showed no change in antigen expression as compared to corresponding unexposed cells (A, C, E, G). Original magnification 1000x
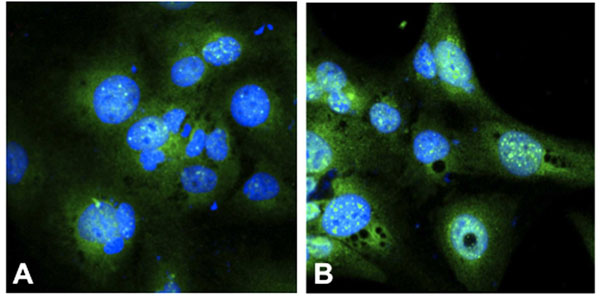
Immunohistochemistry of the glucocorticoid receptor (GR) showed an increase of GR in the nucleus of (B) dexamethasone exposed cells (100 µM) as compared to (A) unexposed cells, indicative of translocalisation of the CG-GR complex to the nucleus. The cell nuclei is stained with Hoechst 33342 (blue), and GR with FITC (green) Original magnification 1000x.
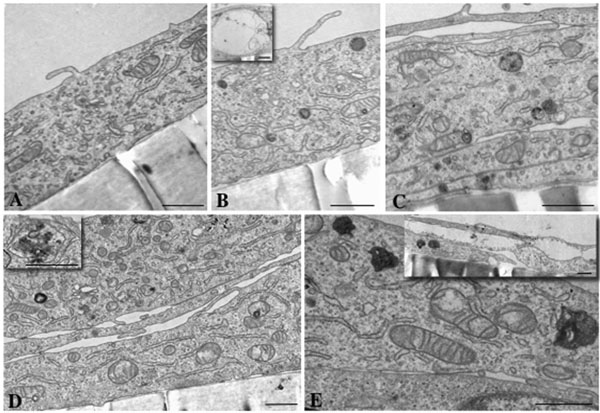
Morphological changes viewed by transmission electron microscopy. A dose-dependent increase in multivesicular bodies in HLEC after exposure to 0.1 µM (B), 1 µM (C), 10 µM (D) and 100 µM (E) dexamethasone for 24 hours was evident. Control cells, 0 µM dexamethasone, are shown in (A). Enlargement of a multivesicular body is shown as an inset picture in (D). Multilayering of cells, present after 1, 10 and 100 µM dexamethasone exposure, is shown in (C), (D) and (E). Vacuoles also occurred, being more frequent in number and size with increasing concentration (see inset in picture B and E). Scale bar = 1 µM.
No significant change in, γ-catenin or vimentin expression was present (Fig.1). Vimentin was used as positive control for adhesion and β-actin was used as loading control in the GR experiments. E-Cadherin was not detectable.
Immunohistochemical Analysis
Immunohistochemical analysis showed a declined expression of N-cadherin and α-catenin (Fig.3B,D) in HLECs exposed to dexamethasone as compared to the unexposed cells (Fig.3A,C). No change in antigen expression of dexamethasone exposed cells labelled with anti-β-catenin or anti-γ-catenin (Fig. 3F,H) as compared to the unexposed cells (Fig.3E,G) was evident.
Translocation of the GC-GR complex to the nucleus was present to a larger extent in the dexamethasone exposed HLECs (Fig. 4B) as compared to the unexposed cells (Fig. 4A). Immunostaining for E-cadherin was negative (not shown).
Morphological Changes as Viewed by Transmission Electron Microscopy
Control HLEC grew as monolayers on the filters. The cells displayed numerous mitochondria with regularly spaced cristae, well developed and branching rough endoplasmic reticulum, and rather prominent and vesicle rich Golgi regions (Fig. 5A). HLEC exposed to 0.1, 1, 10 and 100 µM dexamethasone exhibited dose-dependent morphological changes consisting of vacuole formation and increasing numbers of multivesicular bodies. The latter were most numerous and irregularly shaped in high-dose dexamethasone cells. They contained a very electron-dense material including packed membranes and numerous vesicles. Interestingly, an apparent rearrangement of membrane into dense whirls was seen in association with some mitochondria (Fig.5B-D). At 1, 10 and 100 µM, multilayering of cells was also evident (Fig. 5C-E).
DISCUSSION
Glucocorticoids are frequently used in clinical practice because of their anti-inflammatory and anti-immunologic actions to treat diseases such as allergies, rheumatic and autoimmune conditions.
Glucocorticoids have many diverse effects, including potentially harmful side effects such as causing lens opacities in the posterior pole of the lens (posterior subcapsular cataract, PSC). However, the mechanism behind these glucocorticoid-induced opacities is yet not elucidated. In this study, we examined effects of dexamethasone on cell adhesion, migration and glucocorticoid receptor binding.
We found a decrease in the adhesion molecule N-cadherin as well as decreased expression of α-catenin and β-catenin, adhesion molecules linking cadherin to the actin cytoskeleton, indicating a reduction of the cell-cell binding capacity, which could lead to altered cell migration. A study by Ferreira-Cornwell et al, showed that when N-cadherin was blocked, α-catenin and β-catenin expression were also decreased, while γ-catenin (plakoglobin) was upregulated to compensate for the loss of N-cadherin, α- and β-catenin [20]. In our study, γ-catenin expression was unchanged in cells exposed to dexamethasone as compared to the unexposed cells while exposure to dexamethasone decreased N-cadherin, α- and β-catenin expression. However, the downregulation of N-cadherin, α- and β-catenin in our study may not have been as pronounced as in Ferreira-Cornwell’s study which could explain the difference in ã-catenin expression between the two studies.
However, we could not detect any E-cadherin expression by western blot or immunohistochemistry. Studies in other species or in transfected human lens epithelial cell lines have detected E-cadherin in the lens [30-33]. In contrast, as in the present study, Nishi et al. did not find E-cadherin in native human lens epithelial cells [34]. The discrepancy in E-cadherin expression may be due to different species and transfected versus native cells.
Several studies have verified a functional GR in the lens [6, 10, 11, 23, 24, 28] and that the changes in signalling pathways (MAPK and PI3K/AKT) in the lens are due to specific GR activation [23].
When GC binds to the GR, the GC-GR complex is translocated from the cytoplasm to the nucleus. In the present study, the nuclear fraction of the cell lysate was removed, so that only non-liganded GR in the cytoplasm was measured. We found a downregulation of GR expression indicating GC-GR complex binding, hence demonstrating an active glucocorticoid receptor.
Some studies propose that most glucocorticoid-related effects are directly or indirectly mediated by GRs [9, 11, 23, 24, 28], whereas other studies suggest that glucocorticoids mainly exert their actions through receptor-independent mechanisms [4, 5, 9, 21].
An interesting feature of dexamethasone-exposed HLEC was the dose-dependent accumulation of membrane-delimited electron-dense material found in the cytoplasm. These multivesicular bodies have features of autophagosomes in which vesicles that may represent membrane-wrapped organelles were found. The electron-dense content of these inclusion bodies may also be due to aggregated proteins resulting from protein-steroid adduct formation, since conformational changes of lens proteins due to binding of steroid adducts to lens proteins have been implicated in the formation of the steroid-induced cataract [4]. However, this cataractogenic mechanism has been questioned [3, 8, 35].
Transmission electron microscopy also demonstrated the occurrence of multilayered cells after exposure to 1, 10 and 100 µM dexamethasone. In the cells exposed to lower concentrations or in unexposed cells, no multilayering was evident. The appearance of multilayered HLEC after dexamethasone exposure is indicative of a dysregulation of proliferation, differentiation or migration of the cells, all of which have been discussed as pathogenic mechanisms behind PSC formation [36, 37]. Since there is a direct link between the prolonged use of steroids and this particular type of cataract, the present findings are of great importance for elucidating the mechanism behind cortisone cataract. Studies have shown that lens differentiation, as well as proliferation are controlled by growth factors in the vitreous humour [38]. Alterations of these growth factors by corticosteroids have been suggested to disturb lens differentiation, providing a possible mechanism for how steroids affect lens fibre formation, thereby leading to PSC [3].
The present study indicates that several mechanisms may be involved in PSC formation. However, whether the aberrant cell migration due to the down regulation of adhesion molecules after dexamethasone exposure is a direct receptor mediated effect or an indirect effect caused by GR modulation of transcription of other genes, is yet to be elucidated. Non-receptor mediated effects may also be involved in the pathogenesis of steroid-induced cataract.
ACKNOWLEDGEMENTS
This study was supported by the Göteborg Medical Society, the Swedish Medical Society, the Swedish Society for Medical Research, Karin Sandquist Foundation, Stiftelsen Handlanden Herman Svensson Foundation, Knut and Alice Wallenberg Foundation, Föreningen de Blindas Vänner and Kronprinsessan Margaretas Arbetsnämnd för Synskadade and Ögonfonden.
CONFLICT OF INTEREST
Declared none.